Ⅰ. Introduction
Ⅱ. Materials and Methods
1. Study design
2. Patients
3. Surgical procedure
4. Outcome measures
5. SEM analysis
6. Statistical analysis
Ⅲ. Results
1. Baseline characteristics
2. Clinical findings
3. Clinical outcomes
4. Radiographic outcomes
5. SEM findings
Ⅳ. Discussion
Ⅴ. Conclusion
Ⅰ. Introduction
The dimensional changes in the alveolar ridges resulting from atrophy, periodontal disease, and traumatic sequelae pose a significant challenge for placing dental implants in cases of insufficient bone volume or inadequate horizontal, vertical, and intermaxillary relationships, which further leads to unfavorable functional and esthetic outcomes. Bone augmentation techniques must be considered to achieve a long-term survival and success rate for dental implants.1, 2, 3
Guided bone regeneration (GBR) is the most well-documented and commonly used technique for the augmentation of localized alveolar defects.4 A systematic review evaluated that the long-term survival rate of implants following the GBR procedure was 95.7% (range: 84.7%‒100%) irrespective of the type of membrane and grafting materials used.5 GBR achieves osseous regeneration of bone defects by mechanically impeding undesirable soft tissues and selectively allowing osteogenic potential cells, using barrier membranes with or without graft materials.6
Since the introduction of GBR, extensive research has been conducted on various types of non-resorbable and resorbable membranes. Among these, bioresorbable collagen membranes and particulate bone substitutes are the most common routine procedures performed in clinical settings, demonstrating long-term success.4,7 Collagen-based membranes offer advantages such as minimal intervention, excellent biocompatibility, and acceleration of wound healing.8 However, the major drawbacks of this collagen membrane, such as unfavorable mechanical properties, lack of space maintenance, and faster degradation rates compared with non-resorbable membranes, may contribute to the early loss of barrier function.9
To counteract these challenges, these types of membranes require an underlying bone-substitute material to prevent membrane displacement. Nevertheless, compressive forces at the augmented site could lead to the micromovement of the membrane and/or the underlying graft material. In vitro studies have demonstrated that wound closure and flap suturing can cause significant volume changes in the augmented area, particularly in the coronal portion at the implant shoulder level.10, 11, 12 Thus, dimensional changes can be mitigated using fixation pins,13 block-type substitutes,14 and the flap technique.15 There is still an ongoing controversy and debate regarding the optimal selection of biomaterials for GBR applications.4
Various studies have been conducted to develop biodegradable membranes to minimize the limitations of routinely used membranes in the clinical setting.16,17 3D printing is the most versatile technique that employs various biodegradable synthetic polymers through computer-aided design/computer-assisted manufacturing (CAD/CAM) and layer-by-layer processes.18 Using this technology, 3D-printed membranes with controllable pore size, geometry, and porosity can be prepared to enhance bone regeneration.19 Notably, pre-formed 3D-printed synthetic membranes have distinct advantages, particularly in terms of space maintenance and mechanical properties, and are comparable to non-resorbable membranes in promoting bone regeneration. This represents a promising advancement for future GBR procedures.16 A previous in vivo study compared a resorbable 3D-printed polycaprolactone/β-tricalcium phosphate (PCL/β-TCP) membrane with a collagen membrane for horizontal ridge augmentation. The results indicated that the PCL/β-TCP membrane maintained the augmented site without collapse and significantly increased new bone formation, compared with the collagen membrane with particulate bone substitutes.20
This retrospective clinical study aimed to evaluate the clinical and radiographic effectiveness of GBR using pre-formed 3D-printed PCL/β-TCP and collagen membrane for the augmentation of peri-implant dehiscence defects.
Ⅱ. Materials and Methods
1. Study design
This retrospective case-control study was designed to evaluate the clinical outcomes of 12 patients who had undergone GBR surgery with pre-formed resorbable 3D-printed PCL/β-TCP membrane and with collagen membrane. Both groups grafted with same particulate bone substitutes. All surgical procedures were performed by a single experienced periodontologist (J.H.Y.). The study protocol was reviewed and approved by the Institutional Review Board of Jeonbuk National University Hospital (CUH 2023-03-009-002).
2. Patients
Patients who underwent implant and GBR surgery at the Department of Periodontology, Jeonbuk National University Hospital, between August 2020 and February 2023, were considered eligible for the study. The inclusion criteria were: (1) stable physical health and good oral hygiene, (2) presence of a dehiscence-type bone defect after implant placement, (3) conventional GBR technique using exclusively particulate bone graft with either pre-formed 3D-printed PCL/β-TCP membrane or collagen membrane, and (4) cone-beam computed tomography (CBCT) images available immediately after implant treatment and before re-entry surgery without any artifacts. Patients were excluded if they had been treated with (1) staged GBR due to severe atrophic alveolar ridges, (2) additional fixation such as pins or screws used for membrane stabilization, (3) current heavy smokers (>10 cigarettes per day), and (5) uncontrolled systemic diseases and other diseases that could be contraindications for implant and GBR surgery.
3. Surgical procedure
To achieve optimal implant placement at the predetermined positions, virtual planning was implemented for all cases using the R2GATE software (MegaGen, Daegu, Korea). Patients were instructed to rinse with 0.2% chlorhexidine mouthwash before surgery. Following the administration of local anesthetic lidocaine with epinephrine (1:100,000), a midcrestal incision was made on the keratinized gingiva. Sulcular and vertical releasing incisions were then placed on the mesial or distal aspect using blades no. 12 and 15. Full-thickness mucoperiosteal flaps were raised and implants were placed using a patient-specific 3D-printed static surgical guide designed by the implant planning software (R2GATE). All implants (AnyOne®; MegaGen, Daegu, Korea) were placed according to the manufacturer’s instructions, with adequate primary stability. Simultaneous GBR was performed using a demineralized bovine bone mineral (DBBM) (Geistlich Bio-Oss®; Geistlich Pharma AG, Wolhusen, Switzerland), and covered with either a pre-formed resorbable PCL/β-TCP membrane (LT6 Membrane™; MegaGen, Daegu, Korea) (PM group) or a collagen membrane (Ossix Plus®; Datum Dental Biotech, Telrad, Israel) (CM group). The barrier membranes were trimmed and adapted to the defect size by overlapping at least 2 mm from the adjacent defect borders. Subsequently, all implants were allowed to heal in the submerged position. Tension-free primary closure of the flap was achieved by a periosteal releasing incision using horizontal mattress and interrupted sutures with 5‒0 monofilament absorbable suture material (Monosyn®; B.Braun, Tuttlingen, Germany) and 5‒0 polyglactin 910 absorbable suture material (Vicryl®; Johnson & Johnson, NJ, USA).
After the surgery, the patients were prescribed a course of analgesics (ibuprofen, 200 mg) and antibiotics (amoxicillin, 500 mg) 3 times daily for 5 days. Additionally, they were instructed to use a mouthwash containing 0.12% chlorhexidine gluconate. All patients were recalled for additional postoperative checkups and were enrolled in a maintenance hygiene program. The sutures were removed 10‒14 days after surgery, and re-entry surgery was performed after a mean healing period of 18 weeks. Subsequently, the final prosthesis was delivered 1‒3 months after the re-entry surgery.
The treatment sequence and timeline for this study are illustrated in Fig. 1. Detailed presurgical and surgical procedures and postoperative radiographs are depicted in Fig. 2.
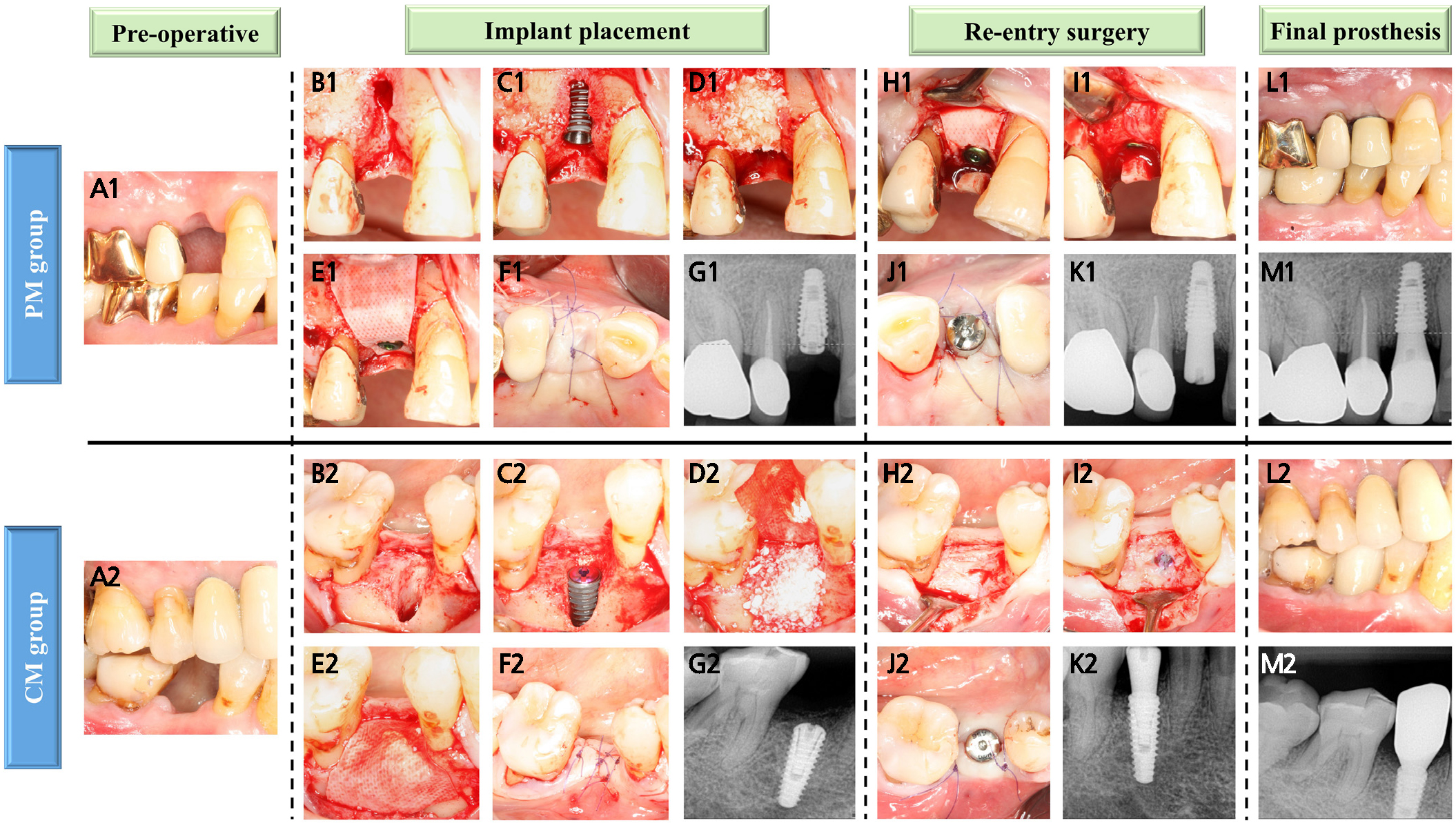
Fig. 2.
Clinical and radiographic images of the PM and CM groups. (A1, A2) Preoperative view of the maxillary right first premolar and mandibular right second premolar, (B1, B2) Dehiscence defect, (C1, C2) Peri-implant dehiscence defect after implant placement, (D1, D2) Bone augmentation with DBBM, (E1) Covered with a PCL/β-TCP membrane and then secured with a cover screw, (E2) Covered with resorbable collagen membrane, (F1, F2) The flap was repositioned and sutured using primary closure, (G1, G2) Periapical radiographs were obtained after implant placement, (H1–J1, H2–J2) Re-entry surgery and removal of the membrane were performed, followed by healing abutment connection, (K1, K2) Periapical radiographs were performed after re-entry surgery, (L1–M1, L2–M2) Delivery of the final prosthesis.
4. Outcome measures
4.1. Clinical outcomes
Defect height (DH) was measured from the implant shoulder to the first bone-to-implant contact (BIC), and defect width (DW) was measured from the mesial to the distal bone crests at the level of the implant shoulder, which were assessed after implant placement and re-entry surgery using a CP 15 UNC periodontal probe (Hu-Friedy, Chicago, IL, USA).
4.2. Radiographic outcomes
To analyze the horizontal bone width (HW) of the augmented region in a direction perpendicular to the long axis of the implant surface toward the buccal aspect of the bone, the following parameters were used: at the implant shoulder (HW0), 2mm (HW2), and 4mm (HW4) apical to the implant shoulder. For vertical augmentation assessment, additional parameters were measured: vertical thickness (VT) and VT at a 45-degree angulation (VT-45). VT was measured along the long axis of the implant, from the level of the implant shoulder to the most coronal portion of the augmented bone. VT-45 was assessed from the oblique line relative to the long axis of the implant at the implant shoulder. Digital radiographic measurements were analyzed and validated using DICOM files through an image analysis software (OnDemand 3D; CyberMed, Seoul, Korea). Bone measurements were performed after implant placement and re-entry surgery by the first examiner who was not involved in the treatment of patients (S.K.). The second examiner (J.H.L.) reviewed the CBCT measurements for all cases by verifying the captured images from the measurement screen. Random CBCT images were selected and measured twice for intra-examiner reliability analyses (intra-class correlation coefficient = 0.94 with 95% confidence interval = 0.86‒0.98).
5. SEM analysis
The surface morphology of 3D-printed pre-formed PCL/β-TCP membrane and collagen membrane was examined using field-emission scanning electron microscopy (Nova NanoSEM 450; Thermofisher, USA) at 10 kV under 70×, 200×, and 500×.
6. Statistical analysis
The results were expressed as the means, standard deviations (SD), and 95% confidence intervals (CI). Data normality was checked using the Shapiro-Wilk test. The clinical and radiographic outcomes were assessed using the non-parametric Mann–Whitney U test to detect the differences between the PM and CM groups at baseline and re-entry surgery, as well as the changes between baseline and re-entry surgery within the group. All statistical analyses were performed using SPSS Statistics software (SPSS Ver. 29.0; Chicago, IL, USA), and a p-value of ≤ 0.05 was considered statistically significant.
Ⅲ. Results
1. Baseline characteristics
A total of 12 patients were included in this study, including 10 men and 2 women. The patient’s mean ages were 60 ± 4.52 years for the PM group and 59.2 ± 13.8 years for the CM group. The demographic data of the study group are summarized in Table 1.
Table 1.
Patient demographic data analysis
Characteristics | PM Group | CM Group | Total (N) |
Sample size | 6 | 6 | 12 |
Sex | |||
Male | 5 | 5 | 10 |
Female | 1 | 1 | 2 |
Age (y) | |||
Mean ± SD | 60 ± 4.52 | 59.2 ± 13.8 | |
Age range | 54‒66 | 37‒72 | |
Reason for extraction | |||
Periodontitis | 5 | 3 | 8 |
Unknown | 1 | 2 | 3 |
Peri-implantitis | 0 | 1 | 1 |
GBR location | |||
Maxilla | 4 | ||
Anterior | 1 | 0 | |
Posterior | 3 | 0 | |
Mandible | 8 | ||
Anterior | 0 | 0 | |
Posterior | 2 | 6 | |
Implant length (mm) | |||
8.5/ 10/ 11.5 | 0/ 5/ 1 | 1/ 3/ 2 | 12 |
Implant diameter (mm) | |||
3.3/ 4/ 4.5/ 5 | 1/ 4/ 0/ 1 | 0/ 3/ 1/ 2 | 12 |
2. Clinical findings
The average healing period was 18.33 ± 1.87 weeks, with no severe adverse effects observed in either group. Additionally, no soft tissue dehiscence, inflammation, gingival swelling, or membrane exposure was observed.
3. Clinical outcomes
The DH and DW measured after implant placement were 3.24 ± 1.35 mm and 4.01 ± 1.25 mm in the PM group and 4.13 ± 2.58 mm and 4.15 ± 0.70 mm in the CM group, respectively. At re-entry surgery, the remaining DH and DW were 0.27 ± 0.40 mm and 0.79 ± 0.92 mm in the PM group and 0.63 ± 0.62 mm and 1.23 ± 1.15 mm in the CM group. No significant differences were observed between the two groups at either time point (p > .05). The changes in DH and DW from baseline to re-entry surgery were −2.97 ± 1.58 mm and −3.22 ± 1.30 mm in the PM group and −3.50 ± 2.89 mm and −2.93 ± 1.20 mm in the CM group, respectively (p > .05). This amounted to DH and DW reductions of 89% and 80% in the PM group and 73% and 71% in the CM group, respectively (Table 2 and Fig. 3).
Table 2.
Bone defect dimensions at baseline and re-entry surgery
Defect dimensions (mm) |
PM Group (N=6) Mean ± SD [95% CI] |
CM Group (N=6) Mean ± SD [95% CI] | p-value |
DH | |||
Baseline | 3.24 ± 1.35 [1.81; 4.66] | 4.13 ± 2.58 [1.42;6.85] | 0.818 |
Re-entry surgery | 0.27 ± 0.40 [‒0.16;0.69] | 0.63 ± 0.62 [‒0.01;1.28] | 0.310 |
Changes in defect height | ‒2.97 ± 1.58 [‒4.63;‒1.31] | ‒3.50 ± 2.89 [‒6.53;‒0.47] | 0.937 |
DW | |||
Baseline | 4.01 ± 1.25 [2.70;5.32] | 4.15 ± 0.70 [3.42;4.89] | 0.699 |
Re-entry surgery | 0.79 ± 0.92 [‒0.17;1.75] | 1.23 ± 1.15 [0.01;2.44] | 0.818 |
Changes in defect width | ‒3.22 ± 1.30 [‒4.59;‒1.85] | ‒2.93 ± 1.20 [‒4.18;‒1.67] | 0.485 |
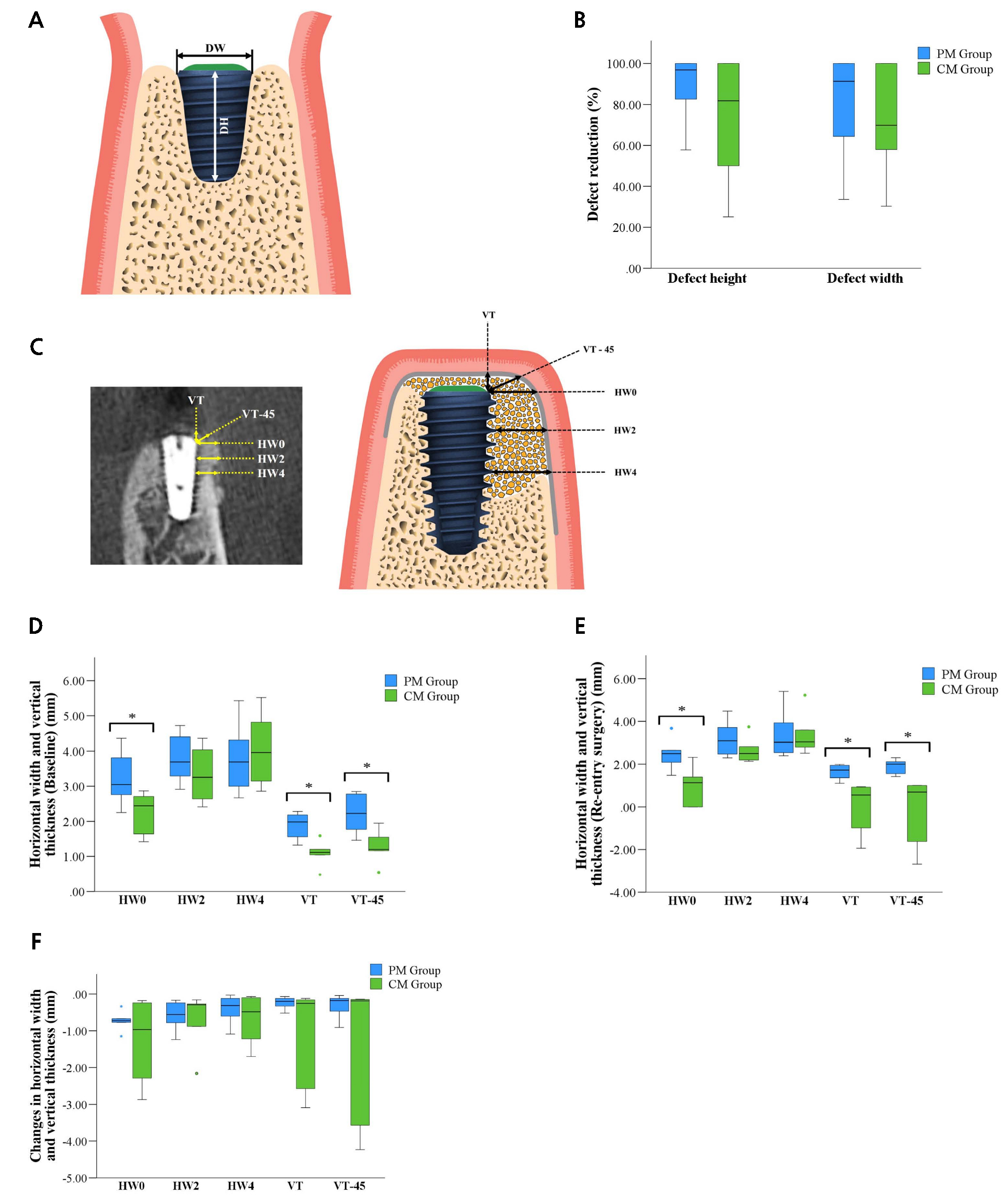
Fig. 3.
(A) Schematic diagram of the measurement of defect dimensions. Defect height (DH) from the implant surface to the first bone-to-implant contact (BIC) and defect width (DW) from the mesial to distal bone crests, (B) Defect reduction (%), (C) Schematic diagram of the measurement landmarks at the buccal aspect of the bone-augmented region at different levels (mm) (HW0–HW4), VT, and VT-45, (D) Box plot results of the PM and CM groups representing the horizontal bone width and vertical thickness of the augmented regions at baseline, (E) Re-entry surgery, (F) Changes in the horizontal bone width and vertical thickness of the augmented region between baseline and re-entry surgeries.
4. Radiographic outcomes
The HW immediately after implant placement for the PM group were 3.21 ± 0.76 mm at HW0, 3.78 ± 0.68 mm at HW2, 3.80 ± 0.99 mm at HW4, while the vertical thickness values were 1.89 ± 0.38 mm at VT and 2.22 ± 0.55 mm at VT-45°. The corresponding values for the CM group were 2.25 ± 0.60 mm, 3.33 ± 0.77 mm, 4.04 ± 1.11 mm, 1.09 ± 0.36 mm, and 1.27 ± 0.47 mm, respectively. Significant differences were found at HW0, VT, and VT-45°(p < .05) between the two groups. After the healing period, the HW changes from the initial surgery were 2.48 ± 0.73 mm at HW0, 3.19 ± 0.89 mm at HW2, and 3.39 ± 1.15 mm at HW4 in the PM group, and vertical thickness values were 1.65 ± 0.35 mm at VT and 1.90 ± 0.34 mm at VT-45° and the corresponding changes for the CM group at the same points were 1.00 ± 0.89 mm, 2.65 ± 0.59 mm, 3.37 ± 0.97 mm, 0.01± 1.20 mm, ‒0.15 ± 1.60 mm, respectively. The significant differences between the PM and CM groups for re-entry surgery were observed at HW0, VT, and VT-45° (p < .05). Conversely, no significant differences were found at HW2 and HW4 between the two groups at baseline and re-entry surgery. The changes between the baseline and re-entry surgery were calculated for all parameters and compared between the PM and CM groups. No significant differences were found in the respective parameters (p > .05) (Table 3 and Fig. 3).
Table 3.
Horizontal bone width and vertical thickness of the augmented region at baseline and re-entry surgery
Measurement Parameter |
PM group (N=6) Mean ± SD [95% CI] |
CM group (N=6) Mean ± SD [95% CI] | p-value |
HW0 | |||
Baseline | 3.21 ± 0.76 [2.42;4.01] | 2.25 ± 0.60 [1.63;2.88] | 0.041* |
Re-entry surgery | 2.48 ± 0.73 [1.72;3.25] | 1.00 ± 0.89 [0.06;1.94] | 0.009* |
Changes between baseline and re-entry surgery | ‒0.73 ± 0.26 [‒1.00;‒0.46] | ‒1.25 ± 1.16 [‒2.47;‒0.04] | 0.937 |
HW2 | |||
Baseline | 3.78 ± 0.68 [3.07;4.50] | 3.33 ± 0.77 [2.52;4.13] | 0.310 |
Re-entry surgery | 3.19 ± 0.89 [2.26;4.12] | 2.65 ± 0.59 [2.03;3.27] | 0.394 |
Changes between baseline and re-entry surgery | ‒0.59 ± 0.40 [‒1.01;‒0.17] | ‒0.68 ± 0.77 [‒1.48;0.13] | 1.000 |
HW4 | |||
Baseline | 3.80 ± 0.99 [2.76;4.83] | 4.04 ± 1.11 [2.88;5.21] | 0.699 |
Re-entry surgery | 3.39 ± 1.15 [2.18;4.59] | 3.37 ± 0.97 [2.34;4.39] | 1.000 |
Changes between baseline and re-entry surgery | ‒0.41 ± 0.39 [‒0.82;0.00] | ‒0.68 ± 0.66 [‒1.37;0.02] | 0.589 |
VT | |||
Baseline | 1.89 ± 0.38 [1.49;2.28] | 1.09 ± 0.36 [0.71;1.46] | 0.009* |
Re-entry surgery | 1.65 ± 0.35 [1.28;2.01] | 0.01 ± 1.20 [‒1.25;1.28] | 0.002* |
Changes between baseline and re-entry surgery | ‒0.24 ± 0.16 [‒0.41;‒0.07] | ‒1.08 ± 1.37 [‒2.51;0.36] | 0.394 |
VT-45 | |||
Baseline | 2.22 ± 0.55 [1.65;2.79] | 1.27 ± 0.47 [0.77;1.76] | 0.015* |
Re-entry surgery | 1.90 ± 0.34 [1.54;2.26] | ‒0.15 ± 1.60 [‒1.83;1.54] | 0.002* |
Changes between baseline and re-entry surgery | ‒0.32 ± 0.33 [‒0.66;0.02] | ‒1.41 ± 1.94 [‒3.45;0.62] | 0.394 |
5. SEM findings
The inner surface of the 3D-printed PCL/β-TCP membrane exhibited a porous, rough architecture compared with the smooth outer surface, resulting in difficulty for cell infiltration (Fig. 4). Moreover, the incorporation of TCP particles was visible on the membrane surface (Figs. 4B to 4G). On the contrary, the surface of the collagen membrane was heterogeneous, with numerous cross-linked fibers and the absence of a porous structure (Figs. 4H to 4J).
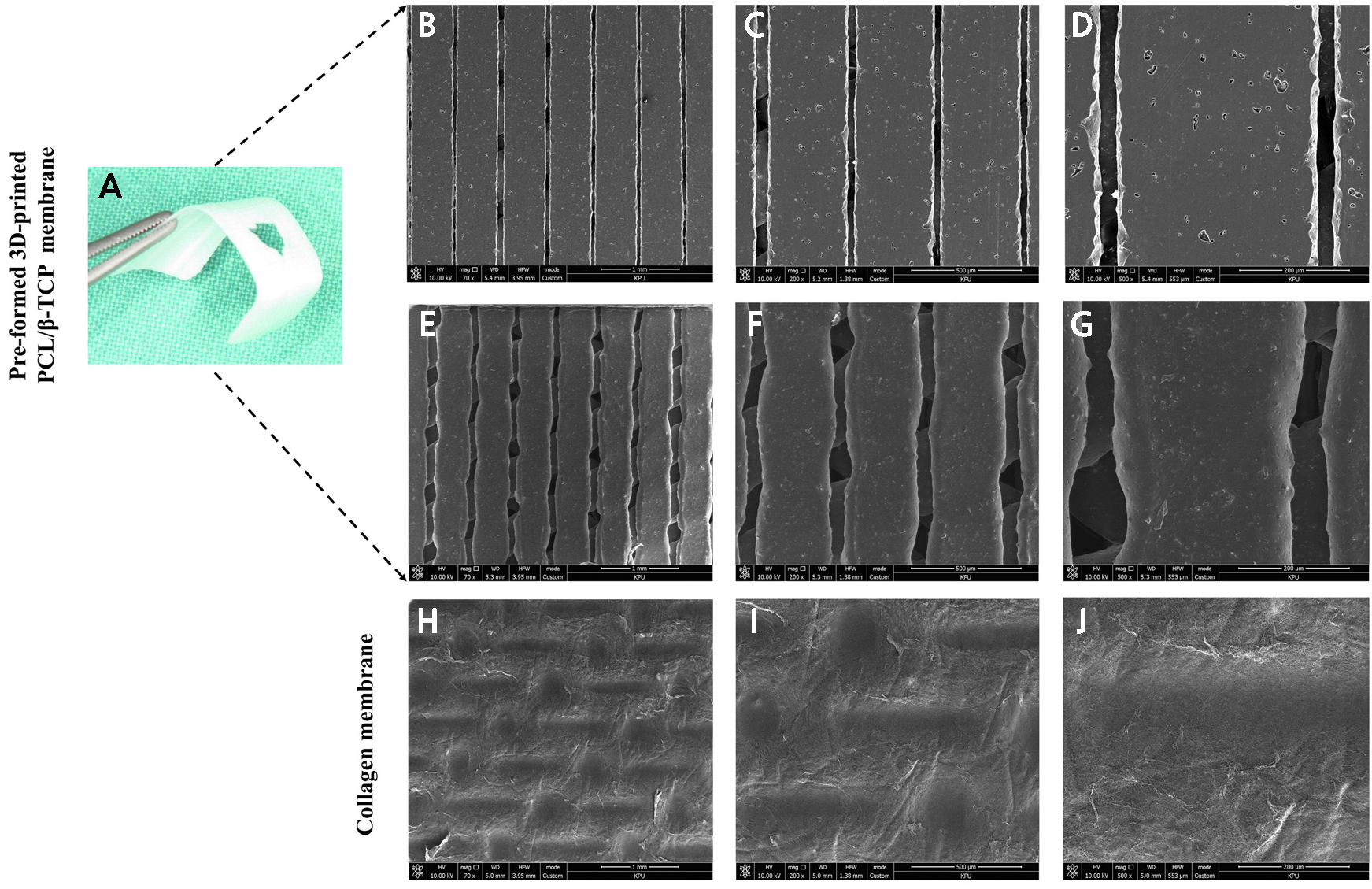
Fig. 4.
(A) Pre-formed 3D-printed PCL/β-TCP membrane used in the clinical setting, SEM images. (B–D) Outer surface toward the soft tissue, (E–G) The inner surface is rough owing to the incorporation of β-TCP particles and a fully interconnected porous architecture, (H–J) SEM view of the collagen membrane densely packed with fibrils revealed a smooth surface and the absence of a porous structure, (B, E, H) Original magnification ×70. Scale bar = 1 mm. (C, F, I) Original magnification ×200. Scale bar = 500 µm. (D, G, J) Original magnification ×500. Scale bar = 200 µm.
Ⅳ. Discussion
The results of the present retrospective case-control study demonstrated that (1) both types of GBR membranes were successful for the resolution of peri-implant dehiscence defects, (2) the mean horizontal bone width and vertical thickness was higher in the PM group, and (3) a consistent decrease in the augmented bone width and thickness was observed in both groups between baseline (immediately after implant placement) and re-entry surgery.
Space creation and maintenance, and membrane stability are crucial requirements for GBR.21 In the present study, the stability of the augmented bone was assessed by measuring the HW on the buccal aspect adjacent to the implants at baseline and re-entry surgery. Both groups utilized the same particulate bone substitutes. The mean HW0 values measured at first surgery were 3.21 mm in the PM group and 2.25 mm in the CM group, exhibiting a significant difference at the level of implant shoulder. At re-entry surgery, both groups resulted in significant reduction in horizontal width, 2.48 mm in the PM group and 1.00 mm in the CM group. This finding demonstrated that the collagen membrane tended to collapse from the augmented site, causing the displacement of the membrane and graft material at the coronal aspect of the implant shoulder. Vertical thickness was measured at VT and VT-45°, and the PM group achieved significantly higher bone thickness than the CM group at the first and re-entry surgery. These results indicate that the 3D-printed membrane maintained volume stability and space maintenance during the overall healing period compared with the collagen membrane.
In addition to the stability of the augmented area, the reductions in peri-implant dehiscence defects in terms of DH and DW were measured at baseline and re-entry surgery in both groups. The DH and DW decreased at re-entry surgery compared with the measurements taken after implant placement. A previous randomized controlled clinical study using a particulate DBBM and resorbable collagen membrane for GBR reported that 85% of buccal dehiscence and fenestration-type defects were resolved.22 In the current study, the mean DH reductions were 89% and 73% in the PM and CM groups, respectively. The mean DW reductions in the PM and CM groups were 80% and 71%, respectively. Although these results were not significant, the PM group outperformed the CM group.
In the present study, peri-implant dehiscence defects were augmented using particulate DBBM and a pre-formed 3D-printed membrane composed of PCL/β-TCP. The combination of PCL/β-TCP improved the mechanical stability, slowed down the degradation rate, and maintained the structural stability required for effective bone regeneration. The addition of β-TCP to PCL improved osteoconductivity and osteoinductivity, reduced the degradation rate of the membrane, and increased the surface roughness.20,23 The SEM findings of the present study evaluated the surface morphology of 3D-printed PCL/β-TCP and collagen membranes. The inner surface of the PCL/β-TCP membrane exhibited an interconnected, rough, porous architecture. Conversely, the outer surface was relatively smooth with small pore sizes, limiting cell infiltration. By contrast, the collagen membrane showed numerous collagen fibers without pore-like structures.
In a previous preclinical study, histological findings reported that the 3D-printed PCL/β-TCP membrane retained the shape of the augmented ridge and exhibited increased new bone formation owing to the space-making ability of this membrane.20 However, the main drawback of this study was the assessment of membrane degradation for only eight weeks, emphasizing the need for extended evaluations to optimize membrane performance over the long term.
The present study has some inherent limitations. First, only a small number of patients were included in the PM and CM groups. Second, the patient’s self-reported questionnaire regarding the severity of pain and swelling was not addressed. Further long-term prospective controlled randomized comparison studies are needed to evaluate the clinical efficacy of the membrane, quantify bone regeneration, and assess the stability of the graft material when placed with these resorbable membranes during GBR procedures.
Ⅴ. Conclusion
Guided bone regeneration using pre-formed resorbable 3D-printed PCL/β-TCP and collagen membrane successfully restored the peri-implant dehiscence defects. The PM group exhibited favorable results in the horizontal and vertical augmented regions, confirming membrane stability and clinical feasibility for successful bone regeneration in peri-implant dehiscence defects.